 |
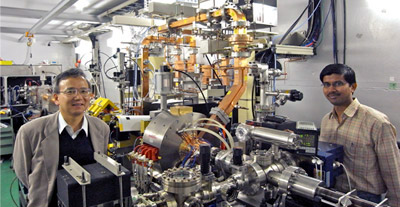
The project leader Prof. Junji Urakawa of KEK (left) and Abhay Deshpande of SAMEER, India (right) standing beside the test laser-Compton X-ray source in the accelerator test facility (ATF) at KEK. The newest feature is the electron gun Deshpande developed (middle) for his doctoral thesis.
|
Your heart should sit on your left side (or on right if you are one in roughly 40,000 people) rather than in the middle. We look pretty much symmetrical on the outside, but when watched closely we realize symmetry is broken in many places in our features.
So it is in the amino acids. Amino acids are the building blocks of proteins, playing vital roles in our biochemistry. The composition of amino acids allows two different arrangements that are mirror images of each other. However, all naturally occurring amino acids take only one of these two forms. What this means is that symmetry is broken in nature. The basic laws of chemistry are symmetric, yet nature chooses one way over the other.
Why the asymmetry? The answer to that question will have a deep implication in the origin of life and even the evolution of the cosmos. One hypothesis is that the asymmetry in amino acids comes from polarization of X-rays that can penetrate well into interstellar clouds. With the hope of proving it, scientists are looking at amino acids with the best quality pulsed, polarized X-rays to date. The study, called circular dichroism, however, will require much faster switching of X-rays polarity than the currently available.
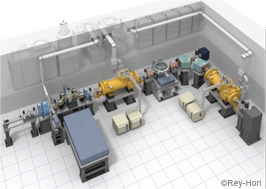
A schematic view of the laser-Compton X-ray source under development.
|
 |
The newly established five-year long Quantum Beam Technology Program is developing tools to help answer these fundamental questions. The aim of the program is to develop a compact and high-quality particle source for commercial use broadly in medicines, life science, information technology, nanotechnology, and quantum science.
The name, Quantum Beam, refers to beams of particles like neutrons, photons, and ions. These particle exhibit quantum mechanical behaviors, and the unique feature of the program is to take advantage of this quantum nature of the particles. Prof. Emeritus Makoto Inoue of Kyoto University announced the program in the kick-off meeting last December to promote the technology transfer broadly to hospitals and research institutions. What he emphasized was the importance of social contributions. The above mentioned circular dichroism measurement in amino acids could possibly bring about major applications to medicines, for example, by improving our understanding of how drugs can be engineered to ease side-effects.
The Quantum Beam Technology Program is a collaboration of around 50 scientists from 18 companies, universities, and research institutions across Japan. Four projects have been approved for the program so far. Led by project leader Prof. Junji Urakawa of KEK is the team of the next-generation compact high-brightness X-ray source project. The collaborators from seven institutions across Japan are now working on research and development of an X-ray source based on a superconducting electron accelerator and high-quality laser cavity.
The superconducting cavity accelerates electrons to an energy of 25 MeV. These electrons hit a tightly focused laser beam and produce X-rays at 0.2-70 keV. This method is called inverse Compton scattering. The device has several different components: an electron gun to fire electrons, superconducting cavities to accelerate and decelerate electrons, a refrigerator to cool the cavities, a laser cavity to store the intense beam of laser, and a beam dump. Expected to be no more than 10 meters in length, the entire station will fit in an average hospital or laboratory room.
"This will be the first application of superconducting radiofrequency cavities to a commercial product," says Urakawa. "Our goal is to make the station compact, and for that superconducting cavities play a significant role."
Superconducting cavities, compared to room temperature cavities, produce a higher accelerating gradient (greater acceleration in the same amount of distance), and are more energy efficient. The team closely collaborates with the KEK-based team of the next-generation 30-kilometer linear collider, the International Linear Collider (ILC), to develop such high performance cavities. The ILC aims at the cavity gradient of more than 35 Megavolts per meter. "For the performance we seek, we need 30 Megavolts per meter, which we've already achieved," says Urakawa. Just a one-meter superconducting cavity will be sufficient to accelerate electrons to the necessary energy.
 |
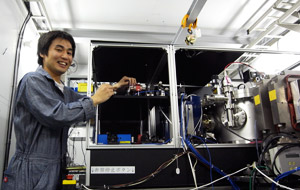
Dr. Kazuyuki Sakaue of Waseda University working on the laser he developed. The iron cylinder on the right is a vacuum chamber that contains an optical cavity (right now only a two-mirror cavity) inside.
|
The present inverse Compton X-ray sources produce about 105 X-ray photons per second. "There are some higher performance X-ray sources such as Helios by Oxford Instrument that goes up to 109 photons per second, but they are generally so expensive that small laboratories cannot afford them," says Urakawa. For the new device, they are aiming for an intensity of 1010 photons per second.
To produce such high intensity X-rays from the beam of electrons, another critical component is an optical cavity for laser. To achieve the target intensity, the team needs to intensify the laser by a factor of a hundred to a thousand. The key to the high intensity is to make the waist size of the beam at the collision point as small as possible, as well as to superpose the pulses as many times as possible. For this, the laser team is developing a four-mirror system that circulates laser pulses which are injected one by one, superposing them in phase to store an amplified resonant laser.
"With four-mirror cavity, we can make the beam much more intense than when we use two-mirror system," explains Dr. Kazuyuki Sakaue of Waseda University. He and members from KEK and Linear Accelerator Laboratory (LAL) in France collaborate to develop the high-performance four-mirror laser cavities.
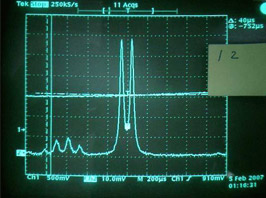
Two-peak signal observed in the laser beam stored in four-mirror optical cavity, showing that the incoming linearly polarized laser beam become circularly polarized as it circulates in the cavity.
|
 |
The interesting feature of the four-mirror laser cavity is that it produces circularly polarized laser beam from the linearly polarized beams of the injected laser. The team uses non-planar configuration for the two concave and two flat mirrors, arranging them in three-dimensional twisted configuration rather than two. This makes the beam's image rotate as it travels the optical path, making the laser circularly polarized. This property can be used to polarize the X-ray photons.
"Changing the polarity of an X-ray beam has been a relatively slow process of changing mechanical position of magnet arrays called undulator," says Sakaue. "If we could instead change the polarity by changing the laser polarization, we would be able to change the laser polarity with much higher frequency."
The current technology with the undulator can alternate the X-ray polarity no more than 10 times per second, while the new technology will be able to change the polarity up to 1000 times per second. This is 10 times more than required to conduct the circular dichroism study of amino acids. Thus, this technology may bring about a revolution in the way we understand biological systems by helping to explain the broken symmetries in biochemistry.
 |
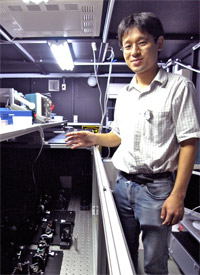
Dr. Yosuke Honda of KEK showing how his laser system works. The picture shows laser system for the compact energy recovery linac (Compact ERL). The compact X-ray source project plans to use the Compact ERL to test the laser-Compton X-rays technique when it starts in 2012.
|
Dr. Yosuke Honda, a member of the KEK laser team, discovered this novel property of the four-mirror cavity and made this technology possible. The discovery came in rather unexpected way. One day last summer, when Honda was working at the four-mirror cavity, he saw peculiar two-peak signal on the oscilloscope (see image). This signal appeared as he was changing the position of a concave mirror. Honda and his team studied what was going on to find that each of the two peaks corresponded to circularly polarized beam. They also made a calculation for the system which successfully explained the experimental data. The team's new idea made it possible to utilize the feature of the four-mirror system for obtaining a stable differential signal to control circularly polarized beam. The published result has rippled out in waves among biochemistry community and beyond.
The team is now working to increase the enhancement factor (the number of pulses that are stored in cavity), as well as to improve the focus of the laser beam. For better enhancement factor, Sakaue has a new plan. The bottleneck is that, for in-phase superposition of a thousand 1-micrometer laser pulses, he needs to adjust the mirror position in sub-nanometer precision. He is now studying a way to utilize a phase modulator that can adjust phase of each pulse before it enters the laser cavity.
The team is also considering an ambitious plan to put the pulse stacking cavity inside a laser oscillator that produce laser beam. "We have some challenges to cope with, but hopefully that will be resolved by the end of the project," says Sakaue.
The object of this project, Urakawa says, is the technology transfer. "I had been doing fundamental sciences, which I'm afraid had not resulted in anything useful to society just because our research stops short for such purpose." Less than one year into this new project, the team has already been making difference. Urakawa and his team are already starting to transfer the technology to universities and companies in Japan. "The result will come out soon. We are also planning to share this technology with Indian institutions." Urakawa says that he would like to see the project through to completion, and witness the affordable, compact X-ray machine installed in hospitals to aid in medical treatment. His three key goals are "safe, useful, and for society."