With the first successful beam commissioning on April 23 this year, T2K's beamline and detectors are making their way to completion. T2K, the Tokai-to-Kamioka experiment, is a unique long-baseline neutrino oscillation experiment that sends neutrino beams across the Japanese archipelago from the Pacific side towards the Sea of Japan. Neutrinos are produced at J-PARC, a high-intensity proton accelerator facility constructed jointly by KEK and the Japan Atomic Energy Agency (JAEA). As the neutrinos travel 295 kilometers underground from J-PARC, they change their flavors in the course of their 1-millisecond flight. The end detector that detects the resulting neutrinos is the world's largest underground neutrino detector, Super-Kamiokande.
Neutrinos are elementary particles with neutral charge, and usually pass through ordinary matter almost undisturbed. They have very small mass, so small that physicists have not yet been able to measure it. There are three known types of neutrinos, or neutrino flavors: electron-, muon-, and tau-neutrinos. There is also an antiparticle counterpart for each. Differences in the masses of neutrinos cause them to periodically change flavor; this is known as neutrino oscillation. The T2K experiment aims to discover the muon-to-electron neutrino flavor change.
Out of six parameters that determine the neutrino oscillation mechanism, there are two parameters yet unknown, referred to as the third 'mixing angle' and 'CP violating phase'. From their previous experiments to look for this type of flavor change, physicists have an upper bound on the size of the third mixing angle. Below this bound is where the T2K experiment will explore by searching for muon-to-electron neutrino flavor change. By comparing the oscillations of neutrinos to the oscillations of anti-neutrinos, T2K's future experiment may be able to examine possible CP (charge conjugation and parity) symmetry violation, which in general could cause the matter-antimatter asymmetry of the universe.
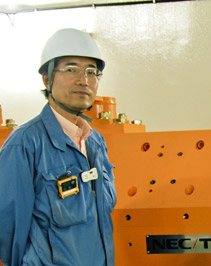
The primary beamline team leader Professor Yoshiaki Fujii of KEK explains how the primary beamline extracts proton beam from J-PARC's main ring.
|
|
T2K's precursor K2K, the KEK-to-Kamioka experiment that operated from 1999 to 2004, used the 12 GeV proton synchrotron at KEK to create neutrinos. Using J-PARC's proton synchrotron, T2K will produce about 100 times more intense neutrino beams than that of K2K. T2K's beamline extracts these intense high-energy protons from the Main Ring at 30 GeV, and guides the proton beam to the graphite target.
The target is a graphite rod 90 centimeters in length. When protons collide with the graphite, pions result. Pions spreading out forward are focused into narrow beams by a series of very high-current electromagnets called horns. The pions then decay into muon and muon neutrino pairs in a 110-meter decay volume, a 3-meter by 3-meter large tunnel (the height gradually increases to 5 meters) filled with helium gas. At the very end of the decay volume, a beam dump absorbs all of the resulting particles except neutrinos and some muons. The resulting clean beam of neutrinos heads to Kamioka at nearly the speed of light.
In April, with the first horn and the target installed, T2K's beamline saw the first beam commissioning. While the intensity of the test beam was only 0.1 percent of the design intensity (3 x 10
14 protons per pulse), the commissioning confirmed successful extraction and transportation of single-bunch proton beams, and also observed clear signals from the pion decay particles, muons, indicating the successful production of muon type neutrinos. In mid-May, the beamline passed the governmental inspection.
|
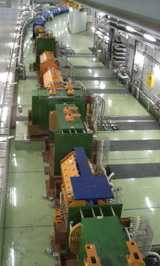
Superconducting magnets (blue magnets at the far end) guide the extracted beam of protons to T2K's final focusing section where the beam is focused to a proper size in normal conducting magnets (green and orange).
|
During the scheduled shutdown from June to September, the T2K teams are installing the rest of the components, analyzing the test data, and making some more modifications to get ready for the next beam commissioning in this Fall with full configuration. One such team is handful of experts who are analyzing newly obtained data at the primary beamline just upstream of the target station. The team constructed magnets and beam monitors in the primary beamline in collaboration with University of Seoul, University of Washington, and Centre d'Etude Nucleaire de Saclay France.
In the primary beamline, proton beams extracted from the main ring take a sharp 80-degree turn to the direction of Kamioka. For this, physicists employ superconducting magnets to create a strong magnetic field in the short, arc region. Generally beamline magnets come in pairs: one magnet to squeeze the beam and one magnet to bend the beam's trajectory. T2K's superconducting magnets, because of limited space and budget, had to combine both functions in one magnet. The magnets, called superconducting combined function magnets (SCFM), combine dipole and quadruple magnets to bend and squeeze at once. These 28 SCFM magnets are the first such superconducting magnets installed in any accelerator in the world. "They had to be designed carefully because it is harder to tune the beam afterwards," explains Professor Toru Ogitsu of KEK, the leader of the T2K superconducting magnet group. "But once it's done successfully SCFM magnets handle the beam much better."
Normal conducting magnets lie in the straight regions in the upstream and downstream of the superconducting magnets. These magnets, designed by KEK's engineer Erina Hirose, have a very high radiation tolerance. The normal conducting magnets in the upstream tune the beam before sending it to the superconducting magnets. In the downstream, "the magnets guide and focus the beam onto the target, while directing the beam slightly downward," explains Professor Yoshiaki Fujii of KEK, the leader of the T2K primary beamline. "Otherwise the beam would simply escape the Earth's round surface without hitting Super-Kamiokande."
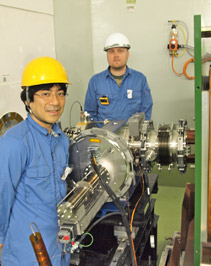
Dr. Nicholas Hastings of KEK (right) and Dr. Hidekazu Kakuno of University of Tokyo at the end of primary beamline around the monitors they've developed. This section of the beamline tilts slightly forward (to the left) so as to send the beam into the Earth.
|
|
Both normal and superconducting magnets need monitors to fine-tune the beams that go through them. There are four types of monitors that physicists have developed for this purpose: those that monitor beam profile, intensity, position, and the beam loss. Beams come in bunches of protons with 600 nanoseconds spacing, and the monitors sitting between magnets measure each parameter in each bunch.
The 19 profile monitors, developed by Dr. Hidekazu Kakuno of Tokyo University, utilize a segmented secondary emission monitor (SSEM), a device that consists of thin metal foil electrodes, to observe the beam profile. When the beams pass through the metal (titanium) foil some protons interact with the foil, producing electrons. By counting the number of these secondary electrons bunch-by-bunch, Kakuno is able to measure the stability of the beam size and the beam center. Though the effect is small, the monitors interfere with the beam causing beam loss in 10
-5 order of magnitude. So each profile monitor has a linear guide mechanism to remove the SSEM from the beam orbit when the SSEM is not in use.
Throughout the design and development, Kakuno and four experts from Mechanical Engineering Center and Cryogenic Science Center at KEK were challenged by the extremely low temperature. Superconducting magnets are kept cool at minus 270 degrees Celsius to keep the superconducting state. Kakuno's monitors, sandwiched between the magnets, need to stay at minus 150 degrees. When he first tested his linear guides, the monitors would not move because the various components shrank differently depending on their materials. "It took three years of testing and making modifications to finally get it work," says Kakuno.
|
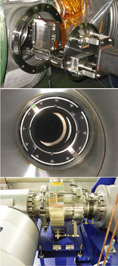
Profile monitor sitting between superconducting magnets (top); beam position monitor for high intensity running (center); and beam intensity monitor between a superconducting magnet and a normal conducting magnet (bottom).
|
Unlike the profile monitor, the intensity and position monitors do not interact with the beam directly. For the intensity monitor, Dr. Masahiro Shibata of KEK, the monitor group leader, uses a current transformer (CT) to measure the magnetic field produced by the beams passing through. Dr. Nicholas Hastings of KEK designed the beam position monitors adopting an electro-static monitor design. The beam loss monitors are completely out of the way sitting well below the magnets simply to catch any radiation coming from the beamline.
The monitor team has finished tests, installation, and calibration. During the commissioning, the team successfully extracted beautiful plots of secondary electron distribution and beam current from the single-bunch beams. Now they are trying to understand the beams by analyzing the data and making modifications to their monitors. "The monitors worked as we expected," says Hastings, "but we found a new background issue." When the profile and position monitors were used at the same location, it seems that the electrons from the profile monitors cause background noise in the position monitor. Hastings is now looking at the problem from two sides; namely, the data analysis side and the physical approach. On the physical side, he has added a solenoid to ward off unwanted electrons.
Apart from Hastings' work to solve the background issue, the team members are working hard to get ready for the next commissioning. They are developing software to share their data with the detector teams both at J-PARC and at Super-Kamiokande. Also, to test the intensity monitor performance with the full intensity beam, the team needs to develop a high current pulser that can produce up to 200A of current to test the linearity of the monitor response. They have confirmed the linearity at currents of up to 90A, and are now working to develop a pulsar with capacitor that can operate at very high voltage to produce the required 200A current.
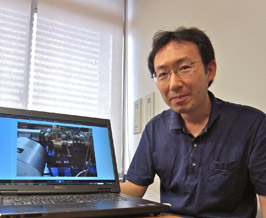
Monitor group leader Dr. Masahiro Shibata at KEK explains how his monitors were assembled.
|
|
"The goal of our monitor team is to guide the beam as precisely as possible during the run," says Shibata. The T2K experiment will start late this year, and collaborators are expecting the first results next year. The project is "challenging but interesting," says Hastings reflecting on many hours in the library, many simulations and many more electronics, " I am looking forward to real particle physics analysis."