 |
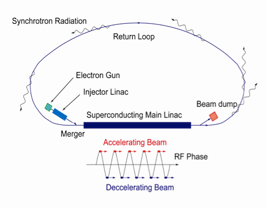
Schematic view of the future Energy Recovery Linac, ERL. Electrons are accelerated to the near speed of light in the main linac, go around the arc, and when they return, the energy is used to accelerate newly injected electron bunches in the linac.
|
The energy recovery linac (ERL) X-ray source could help bring about a new era of materials science. By illuminating a specimen with a short-pulse, coherent, nanometer sized beam of X-rays, scientists will be able to conduct non-destructive measurements on rapidly evolving dynamical materials and organisms with submicron resolution. Multitudes of applications will be available in material, life, chemical and environmental sciences. Particularly interesting examples include: next-generation high-speed communication devices, catalysts for clean hydrogen energy, drug-discovery research, sub-cellular imaging, and efficient use of light energy.
The key to making such measurements is having an X-ray source with short pulse-length, small beam size, and a high degree of coherence. Every chemical structure around us, both living and non-living, undergoes continuous dynamical change at very short time-scales. One good example is the process of photosynthesis. Photosynthetic proteins absorb light and convert the radiation energy to chemical energy in around a hundred femtoseconds (100 times 10
-15 seconds). Current technology cannot produce pulses shorter than tens of picoseconds (10 times 10
-12 seconds). This means the resulting information is a broad average over thousands of femtosecond processes. The nanometer sized, short-pulsed synchrotron radiation from ERL will be able to explore these intriguing processes in real-time, with no averaging.
One special feature of the beam produced at ERL is the coherency of the X-rays. In current X-ray imaging methods, X-ray photons are scattered by the inner structure of a sample when they strike it, creating an interference pattern on the screen behind the sample. Scientists reconstruct the inner structure of the sample from the resulting pattern of bright spots (amplitude information) available at the screen. The phase information, however, is lost. Since the reconstruction method involves moving back and forth between phase space and real space, the lack of phase information is a serious problem, especially when dealing with complex, non-crystalline structures. If the X-rays are coherent and they all strike the object in phase, then scientists can reconstruct the sample's inner structure because they have a clear knowledge of phase.
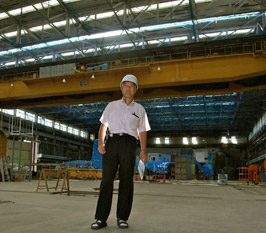
Prof. Hiroshi Kawata, the head of the ERL Project Office, explains how the Compact ERL components will be constructed in the east experimental hall at KEK.
|
 |
"The coherency will allow us to do atomic and nano-scale imaging, which is not so easy even at a third-generation light source like SPring-8 synchrotron in Hyogo because of the low degree of spatial coherency," says Prof. Hiroshi Kawata of KEK, the head of the ERL Project Office. ERL's X-rays will have a high degree of spatial coherency, and a wavefront of the ray can encompass the entire sample all at once. With an intensity roughly two orders of magnitude brighter than other facilities, and a pulse-width roughly two orders of magnitude shorter than other facilities, the ERL will be the world's highest performance X-ray facility.
The ERL will be a 400-meter long linac whose ends are connected by two 800-meter return arcs. The main linac will accelerate injected electrons up to 5 GeV at a current of 10 - 100 milliampere. In the arc region, electrons at 5 GeV produce synchrotron X-rays along the way. These electrons are used only once to keep the X-ray beam clean and short-pulsed. At the end of the second arc, these electrons are not just dumped. Instead, they are used to accelerate a newly injected beam of fresh, clean electrons in the main linac. The ERL team also plans to build an additional linac, a single mode free electron linac (FEL) called XFEL-O, which will drive electron beam further to 7.5 GeV. As well as being the brightest, this X-ray source will have perfect spatial and time coherence.
To understand how the ERL re-uses the energy of returning electrons, you need to understand the mechanism of acceleration in the main linac. For the ERL, superconducting cavities accelerate the electrons. Each cavity is a 9-cell niobium pipe that looks like long-string of donuts. The cells (donuts) are shaped to create an alternating electromagnetic wave with just the right shape and frequency for electrons to ride on the crest of the wave. If, however, electrons ride on decelerating mode instead of the accelerating mode of the wave, those electrons will slow down. The energy extracted from those slowing electrons is used to feed the field to accelerate freshly injected electrons. The used electrons are decelerated to 5 MeV, and then dumped at the end of the main linac.
 |
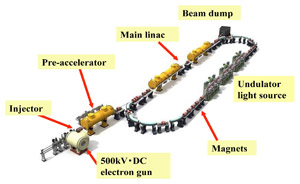
Conceptual figure of the Compact ERL.
|
Reusing the energy of electrons in this way makes the ERL much more energy efficient. For example, consider a beam with 5 GeV electrons and a current of 100 mA. Without energy recovery, the linac would require 500 megawatts of power. With energy recovery, if the electrons are decelerated to 5 MeV, then the linac ideally needs no more than 0.5 megawatts of power. So, is this an energy saver? Kawata answers: "Well, we will drive the 500 megawatt beam with a thousandth of that power, but we still need tens of megawatts to run the accelerator, to cool the superconducting cavities."
While the shape of the cavity allows reuse of energy in the electrons, the superconducting nature of the cavity allows the ERL to produce pulses at an unusually high repetition rate. At a linear accelerator such as the linac at KEK that is based on normal-conducting (high-temperature) technology, there is an upper limit to the acceleration frequency of electron bunch. Electromagnetic fields in the cavity to accelerate the electrons induce wall currents which heat up the cavity wall, causing the energy loss on the cavity wall. For this reason, the repetition rate of electron bunch for such normal conducting accelerator cannot be brought up beyond 50-100 hertz. Since the ERL cavities are superconducting, there is no issue of induced wall currents. Thus, the superconducting ERL will eventually be brought up to a repetition rate of 1.3 gigahertz.
To test these innovative concepts, dozens of ERL collaborators from 9 universities and research institutes (including KEK and Cornell University) are now building an ERL prototype, the Compact ERL. Currently under development at KEK, the Compact ERL will drive electron beam at 60-200 MeV at one-twentieth size of 5 GeV ERL. As well as proving the feasibility of the critical technologies for the full facility, the collaboration will also produce new science using the X-rays produced at the Compact ERL.
Although the Compact ERL will not produce X-rays directly, by colliding electrons with laser beams, the scientists will be able to produce X-rays at 10-50 keV. This small (a few tens of microns) point-source will provide a new imaging technology which can be used to obtain phase contrast images of soft materials. "The X-rays from the laser inverse Compton scattering will capture images of not just the bones but also the soft tissues on your hand," explains Kawata. The use of the laser induced Compton X-ray source can also do some of the same femtosecond science planned at the future 5 GeV ERL facility. Though at low intensity, the study is expected to bring about improvements in time resolution measurement.
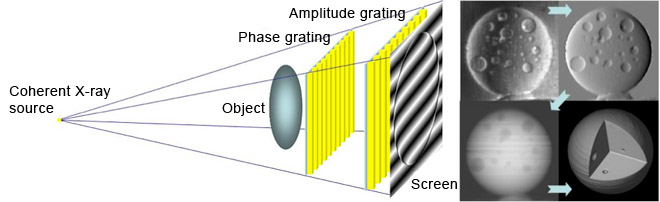
One possible scientific application of the Compact ERL is advanced X-ray imaging using laser inverse Compton scattering. The phase contrast imaging (right) gives a very clear 3-D image, and will be very useful in medical imaging.
(http://mml.k.u-tokyo.ac.jp/indexJP.html)
|
Another use for Compact ERL X-rays involves intense terahertz radiation. When the electron bunch is very short at sub-picosecond in length, the coherent synchrotron radiation wavelength becomes comparably large at around terahertz (10
12 Hertz), so that the synchrotron radiations emitted from electrons in a single bunch appear mostly coherent. Because of the coherency, the radiation amplitudes superpose and become much more intense. The Compact ERL will provide 7-8 orders of magnitude more intense light, in the terahertz region, than currently available at any facilities around the world. Kawata says that this might bring forth a major advancement in semiconductor production process, where selective diffusion of doped atoms using the terahertz radiation may become possible.
"Though there is materials science to look forward to, our main target is to develop the technologies to build the successful 5 GeV ERL," says Kawata. Now the renovation of the east counter hall at KEK is underway. The Compact ERL construction will begin early next year, while the research and development of components such as superconducting cavities, electron guns and lasers will continue until the end of 2011. "The 5 GeV ERL will shed light on heterogeneous systems that exist ubiquitously around us at a sub-nanometers resolution, for the first time in our history. We hope to realize the science at the 5 GeV ERL in the near future."