NEUTRINO OSCILLATION EXPERIMENT
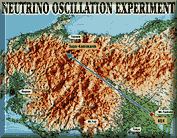
According to the present knowledge of particle physics, the universe consists of six quarks, six leptons, called fermions, and bosons which mediate interactions between them. Protons and neutrons which make all of the normal nucleus have mass about 1 GeV/c2 (10-24 grams). The heaviest fermion observed so far is the "top quark". This particle has mass of about 250 times that of protons (250 GeV/c2). other fermions have mass around 1/1000 to several times that of protons. On the other hand, the lightest fermion known is neutrinos that have mass less than 100-millionth of proton (less than about 10 eV/c2).
Why there are so many fermions?
Ordinary matter (our body, stars, galaxies, etc.) are made of nucleus, which composed of protons and neutrons, and electrons. Nucleus sometime transforms into other nucleus, emitting electron-type neutrino. The essential ingredients of the present universe are four kinds of fermions, i.e., two types of quarks, which make proton and neutron, electron and electron-type neutrino. Other fermions can exist only in high energy environments, such as in accelerator experiments. It appears that other fermions are essentially copies of those four kinds of fermions. However, their masses are different! This is called "generation problem". We simply have no idea why so many fermions exist in nature with different masses.
Why the fermions have those mass values?
This is one of the most fundamental subject in particle physics. We can expect quite reasonably that the studies of neutrino mass will lead us to physics beyond present understanding of particle physics. If the neutrino mass is exactly zero, it deserves a new presently-unknown principle which "demands" the neutrino mass to be zero. (The photon mass is zero because of electric charge conservation.) However, there is no compelling reason for neutrinos to be massless. On the contrary, most of the Grand Unified Theories (the class of theories that try to extend physics beyond present knowledge of particle physics) predict small but finite neutrino mass that would show up at much higher energy than presently reachable.
How we can search for such a small mass? - How neutrino oscillates with time?
In quantum mechanics, physical system (or particle) can be defined by various characteristics (called quantum numbers). If a particle is defined by one quantum number, it is a super-position of the states of other quantum numbers. Obviously mass is one of quantum numbers. Neutrinos have extra characteristics, called electron-type (ne), muon-type (nm), and tau-type(nt). Each type determines what type of lepton is produced by neutrino interaction or what type of lepton is produced with a given neutrino. If neutrino produced with muon, for example, the neutrino is called muon-type neutrino and it produces muon when it interacts with matter. In general, nm is the super-position of various states with given masses. Once nm is produced, each component with given mass propagates in space-time differently, resulting different mixture from the original one. This is called neutrino oscillation. The smaller the mass difference is, the slower the oscillation is. If they travel a long distance, the effect of oscillation would be measurable. Neutrino oscillation studies are thought to be only possible mean to search for a neutrino mass difference well below 1eV/c2.
What kind of mass region we should be searching?
Although no definite theoretical guidance exists for the neutrino mass, there are a few indications that imply a finite neutrino mass. One is the existence of dark matter in the universe. This kind of matter must not interact strongly. Otherwise it can be seen by astronomical observation. Neutrino with finite mass can be a good candidate for such an object. If the dark matter is all due to massive neutrinos, the heaviest neutrino should have a mass greater than several eV/c2. The experiment at 800 MeV proton linac (LSND : Liquid Scintillator Neutrino Detector, Los Alamos), using 30 MeV neutrinos with a distance of 30m, indicated a rather large (several (eV/c2)2) mass difference between nm and ne.
The other indications of neutrino oscillation exist in cosmic ray experiments. The results of Kamiokande and Super-Kamiokande on neutrinos produced in the atmosphere provided strong hints that neutrinos oscillate during the propagation between the production in the atmosphere and the detection on the ground level. The atmospheric-neutrino results clearly show that the nm flux is decreased relative to that of ne. The oscillation length in the atmosphere is from 10 km to10,000 km with the neutrino energy of the order of 1 GeV. This parameter region can be explored with a high intensity neutrino beam from a proton accelerator and with massive detectors.
The other possible neutrino oscillation phenomenon is the solar neutrino deficit. The results indicate that a significant fraction of the ne from sun is lost or transformed into other type of neutrinos, which interact with less interaction cross section or do not interact at all in the detector. These three experimental results give the following three distinct mass differences;
(1) LSND (nm and ne ) : several (eV/c2)2) ,
(2) Atmospheric neutrino (nm and nx) : about 0.01 (eV/c2)2),
(3) solar neutrino (nm and nx) : about 0.00001 (eV/c2)2).
since we know there exists three types of neutrinos, there should be two mass differences. This implies that at least one of the experiments is giving incorrect answer or that a new kind of neutrino exists. We believe that it is now of utmost importance to further study the neutrino oscillation phenomena with well defined accelerator neutrino beams.
Advantages of the Experiment
The advantages in carrying out the long baseline neutrino oscillation experiment combining KEK-PS and Super-Kamiokande is summarized as below.
Fine-grained detector (Near Detector 2) :
Four-ton fiducial mass immediately after the 1k-ton water Cherenkov detector can be used in analysis. The detector consists of water tubes with scintillation fiber sheets for tracking charged particles, scintillators for timing measurement, lead glass blocks for electron energy measurement, and iron with drift chambers for muon energy measurement. The main purpose of this detector is to measure flux, spectrum, and composition of nm and ne in the neutrino beam. The detector can measure the muon momenta up to about 3 GeV/c and can define the fiducial volume with a resolution much higher than that of the 1k-ton detector.
Super-Kamiokande detector, The Far Detector :
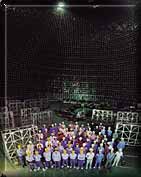
The fiducial mass will be 22,000 ton and the distance is 250 km. This is the main detector to observe neutrino oscillations in the small Dm2 region. The position of the detectors has been measured with the Global Positioning Satellite (GPS) system with an accuracy of less than 1m. Thus it is easy to align the whole detector system to much better than 1-mrad.
The KEK-PS produces an intense nm beam with very small ne contamination ( < 1% ). The energy of the nm beam peaks at about 1 GeV and extends to 4 GeV, with an average energy of 1.4 GeV. In this energy region, the alignment of the beam line and detectors is not critical due to a rather large pion decay angles. For example, a 1 mrad mis-alignment of the central axis of each component induces less than 1% reduction in the neutrino flux. We expect more than 40,000 neutrino events in the 4-ton fiducial volume at the detector in twelve months, giving a good monitoring. We expect about 400 neutrino events in the 22,000-ton fiducial volume of the Super- Kamiokande detector, if there is no oscillation. If the results of an atmospheric neutrino observation are due to a neutrino oscillation, we expect large reduction of the number of events. These numbers are certainly large enough to definitely confirm the atmospheric neutrino results.
Present status
KEK PS Beam Line: For the fast extraction of 12 GeV proton beam from KEK-PS, the fast kicker magnets are being constructed. The system will be installed in summer of 1998. The installation of magnets for 12 GeV proton beam will be completed by the end of 1998. Other critical items of neutrino beam is the target and horn system. The horn system consists of two horns. The horn system has been constructed and tested extensively. The photograph on opposite page shows the newly constructed horn to be used in the experiment.
Near-Detector: We have modified and moved the existing 1,000 ton detector previously used for other experiment.
The entire near-detector will be installed by the end of 1998 and we are planning to start data taking in January 1999. This schedule makes KEK-Super-Kamiokande long baseline neutrino oscillation experiment to be the first accelerator experiment in the world, exploring the Dm2 around 10-2 eV2 region. And we can attain enough sensitivity by the year 2001, if the accelerator can deliver 6x1012 protons on target per each spill for total of 12 months of beam time.